What Even is Quantum?
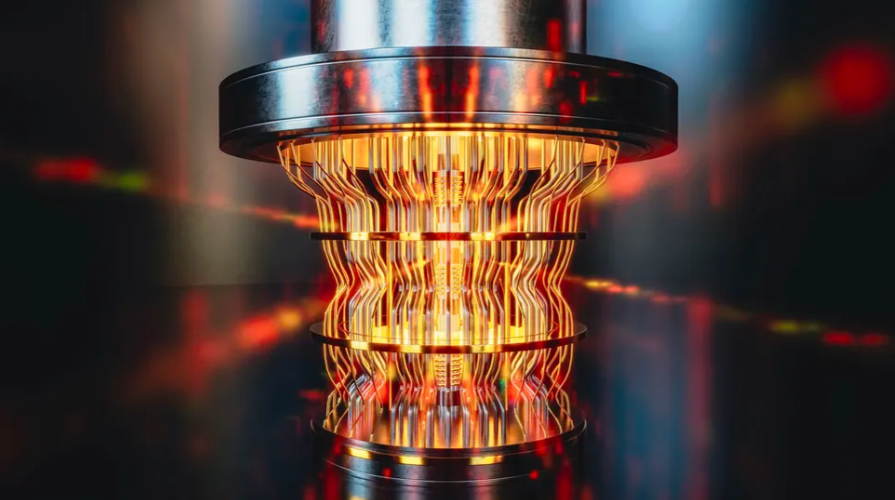
Have you ever seen a Marvel movie? Every time they demonstrate advanced weaponry and technology that they have no clue as to the innerworkings of - "Quantum!" is their safe word. In the modern zeitgeist, it's become a catch-all term for any tech that seems impossible, futuristic, or overly-powerful. But in the real world, beyond the realm of superhero movies, "quantum" computing isn't just a plot device. It's a technology that could change everything. Pursued to its potential, it is the herald of the new computing frontier! But what are the real-world challenges and possibilities that lie ahead?
The Wizard Behind the Curtain
Let us first begin by addressing cultural myths, as it is initially simpler to dispel them then rush straight to the complexity of what quantum technology actually is. First off - no, quantum computers are not "almost here" - at least in terms of scale. They are nowhere near ready to replace the household PC as a mass-produced device for all. Guaranteed, if you're reading this, you will never in your lifetime line up at the Apple store to purchase the first "quantum iPhone." Quantum technology is still in its earliest stages of development, presents massive challenges that stump even the brightest scientists of our time, and in hardware - makes even the highly-sophisticated classical machine look like a Lego set. Importantly, even when we arrive at such a day where quantum computing plays a major role in society, it will not solve every problem. It will eventually target the most complex of computational demands - tasks such as convoluted molecule simulations and interactions, and cracking AES-256 encryption (and beyond) in a matter of seconds. However, it's still not magic - though in understandability, it very well may be. The innerworkings and computer science at play in quantum are incredibly difficult to grasp. However, quantum technology literacy in itself is not reserved just for the geniuses, the same way that literacy of AI and the engineering of it are vastly different beasts.
It must be drilled - a quantum computer is not a "super-powered" computer. Often, a quantum machine in concept is regarded as a much faster and more powerful version of the classical machine we've grown accustomed to. In truth, they operate fundamentally differently down to the very hardware, are currently incapable of the convenient PC interface we've come to know and love - and as the name would suggest - they inherently utilize quantum mechanics to function. Quantum mechanics, in its most user-friendly reduction, is the realm of science in which the familiar and trusted laws of classical physics break down. At the atomic and subatomic levels, though classical physics has proven highly reliable - it has proven an incomprehensive, though by no means "shabby," interpreter of reality. The behavior of matter itself at the most granular levels we can observe presents tendencies that are counterintuitive and paradoxical to the rules as we know them, thus quantum computing was born - not to reinvent the wheel, but to construct a hovercraft, per se.
In addressing these present snags and paradoxes, quantum science stipulates the concept of superpositioning: a particle's ability to "be in multiple states" simultaneously. To explain: at the most foundational level, the classical machine utilizes units known as bits. These bits may either be 0 or 1, simple. Quantum computing contrarily utilizes the qubit, which is... complicated. On the topic of qubits in particular - stay off the forums, there is some very noisy misinformation spreading around. Perhaps the most important clarification this post could make - the statement that a qubit is "both 0 and 1 at the same time" is oversimplified to a fault, and highly misleading - and a proper understanding of the implications of superpositioning is paramount to remedy the risk of taking that statement at face value.
So, imagine you spin a coin. Prior to the inertia wearing off and gravity prevailing, the coin is neither heads nor tails. It exists in an ambiguous and arguable space. A qubit is comparable, heads being 0 and tails being 1, and the coin itself being unbound by any such comparable laws of force and gravity that would decide its landing. Paradoxically, however, the development of superpositioning theory is halted barely a step off the porch - though its prevalence is evident. This is because there is no way we know how to measure the position of the qubit itself without effectively collapsing this postulated superposition - and it ironically serves us right for demanding boolean answers of a force of nature which is fully-unburdened to comply. This is to say, when the qubit is measured, yes, the answer can only ever be 0 or 1. Given that, how does it not directly assassinate the entire superpositioning theory? Because when you measure qubits a series of times, the results are inconsistent. Toto, I don't even know what these are, but we're certainly not working with bits anymore. Whether or not this implies that a qubit is in a state of constant motion between 0 and 1, or rests in an amorphous space between 0 and 1, is a vital question demanding of an entirely new measurement paradigm. We can however state, given what we do know, that a qubit has a certain probability of reading as 0, and a certain probability of being 1. Those probabilities are in constant flux and shift to the very nanosecond of reading, and while we know how to "measure" the qubit like a bit (which is clearly unideal), the measurement of these probabilities has proven incredibly elusive. Accordingly, the true metric by which the qubit ought to be measured is yet to be innovated, halted by this stump.
Linear Approaches to a Non-Linear Reality
Grasping the foundations of quantum computing is all quite exciting, quite complex, and quite like cardio for the brain - but what makes it exciting? With our widespread classical computing machines, which are powerful in their own right, what pushes the envelope to indulge quantum development? Honestly, classical computers, with all their sophisticated capabilities, are reaching their limits sooner than later in the rapidly-developing world of tech. They are now increasingly more often being confronted with problems bearing complexity the classical machine is unequipped to solve. Especially in the spaces of interaction simulations in the pharmaceutical sector, optimization of Big Data workloads past the petabyte, and cracking the most powerful encryptions, quantum will flip the entire paradigm.
In fact, 128-bit encryption was long-considered suitably unbreakable, and still technically is, as we have no evidence that quantum computing has developed far enough to successfully break it. What we do know, though, is that despite 128 still being considered iron-clad, 256-bit encryption has quickly become the new standard at the enterprise level. This security upgrade is not arbitrary, it poses extra costs and maintenance procedures to these firms, who gladly pay regardless - as they are not confident in the extent to which the encryption will hold against mysterious quantum capabilities. The classical machine would take approximately 2.29(10^32) years to crack an AES-256 encryption - the number is so monstrous it can't even be written, but it's considered several millions times the age of the known universe. Quantum however stands scarily better chances - it's postulated that 256-bit encryption is more-or-less going to be suitable until at least 2050 - but that statement's been highly subject to debate. After all, every lock is unbreakable - until it isn't. The progress, much like the innerworkings, of quantum computing itself is decidedly non-linear, and makes such an upgrade necessary in the face of uncertainty. The possibility that this tech could exponentially level up on us overnight is the first thing we can confirm is not fictional.
The classical machine soars at linear, iterative tasks. Imagine a truck driver: considering all possible routes and traffic conditions for their delivery. The classical computer will check every single route one by one in isolated fashion, even if very quickly, becoming inevitably more inefficient as the number of possibilities increases. However, the driver will still arrive at the most efficient computed route rather quickly, and the classical paradigm will still be highly useful in this case. So then - to scale up the intensity of the probability quickly - what happens in pharma when vast genomic datasets are analyzed to render disease mechanisms? Furthermore, what happens when this data is leveraged to form personalized treatments with trial drugs for which there is little information but assumed high potential of success? The tiniest disparities in patient attributes will determine appropriateness of treatment - let alone any medications they could already be taking, other illnesses they may be combating, lifestyle, and so on. If a classical computer could shed tears, that would do it - good luck, that problem would outlive us both.
It is in such cases where quantum computing, rooted in an even arguably anti-linear logic, changes the game. Whereas at its very best, the classical machine can perform numerous tasks in a row at lightning speed - the quantum machine never needed a "row" to begin with. This non-linearity is a direct consequence of the principles of quantum mechanics, particularly the superpositioning we've discussed. If 0 turns the lights off, and 1 turns them on, the qubit may scale the dimness. This allows quantum algorithms to explore multiple possibilities simultaneously, providing a significant computational advantage. It will explore as many scenarios as possible simultaneously - limited only by the power of the hardware provided, but a "line" or "agenda" of tasks is not natural to a quantum machine. Speaking of its hardware, next we'll dive into the surprising physical realities of a quantum system.
Some Like it Hot. Not Quantum
Scroll back up to the top. Look at that photo. If you're like me, you'd assume that that machine is extremely hot. And you'd be woefully incorrect. That machine is actually creating a powerful closed-loop cryogenic field, housing the closest temperature to absolute zero the human race has ever been able to produce! For reference:
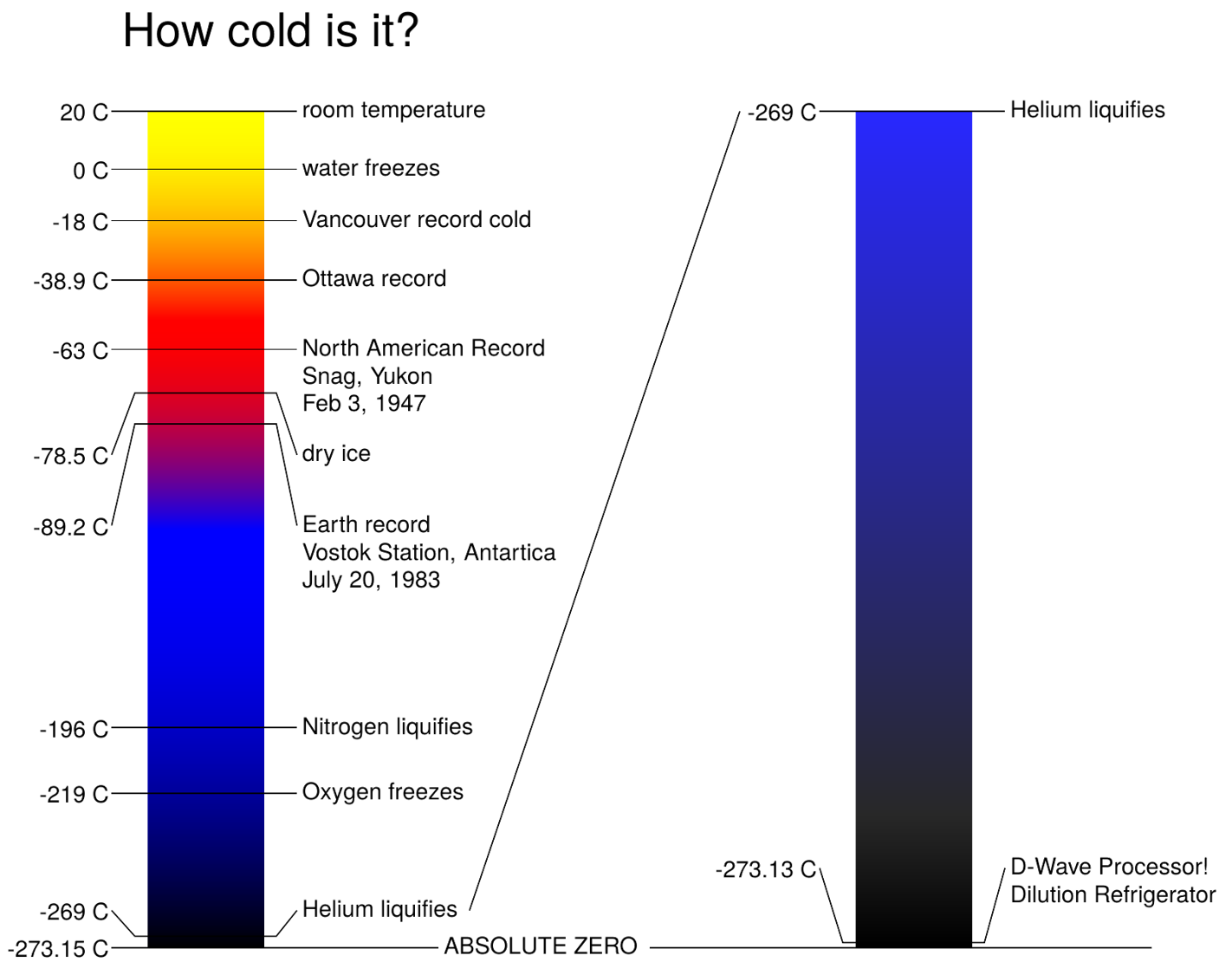
The D-Wave Processor Dilution Refrigerator is one of the most advanced dilution refrigerators ever created, and these machines are the key ingredient in making quantum computing possible at all. To translate the temperature listed above in Celsius, the D-Wave is capable of reaching 0.02 Kelvin, a temperature 180x colder than interstellar space. For reference, 0 Kelvin is the theoretically impossible temperature at which matter itself ceases. These incredibly cold temperatures enable the superconductivity that quantum systems demand. Superconductivity is the state of matter in which materials exhibit zero electrical resistance, and superconducting materials are the building blocks of the qubit itself. In other words, they make it so extremely cold that electrons move in a very slow, highly-coordinated, collective way - allowing for precise control of quantum information. This unique state also exhibits the Meissner effect, where magnetic fields are completely expelled from the superconductor, creating a magnetically shielded environment crucial for maintaining the delicate quantum states of qubits. Even more impressively, IBM is currently in the process of constructing the fabled Goldeneye refrigerator - which is aimed to be even colder, and meant to be capable of housing the world's first 1M-qubit processor! These achievements and aspirations seem like a work of fiction, yet here we are.
Dilution refrigerators achieve this feat with helium isotopes He-3 and He-4, which contain different unique quantum properties. The simplest way to explain would be that He-3 atoms are "more energetic" at extreme low temperatures in comparison to He-4 - and when these two isotopes make contact, there is an energy transfer from He-3 to He-4. Ironically, this sensation is deemed the "heat of mixing" effect, though heat would appear contrary to its very meaning. This transfer ultimately results in a decrease of the overall temperature of the mixture. To understand this, we must first understand heat capacity.
Heat capacity is the measure of how much energy is required to raise a substance's temperature by a certain amount. He-3 (the "more energetic" one) possess a heat capacity lower than He-4 (the "colder" one). What this actually translates to: they do not cancel out - when they make contact, He-3 will cool down more significantly than He-4 will heat up. Then, the "heated" He-4's are continuously removed from the environment by the dilution refrigerator's design. Even though the cold would win this one regardless, this empowers the cooling process even further. Imagine a hot cup of coffee placed in a large, cold container. The coffee will lose heat and cool down, while the container will warm up slightly. But the overall temperature of the mixture will still decrease, because the container is so much larger than the coffee cup. Dilution refrigerators use a similar principle, where He-3 loses heat to He-4, but the constant removal of the warmer He-4 results in a significantly magnified overall cooling effect - which cannot be as simply analogized. The result is the namesake of the mechanism, the dilution, which plays the most crucial role. He-3 is diluted in a larger mixture of He-4, which results in the He-3 molecule dropping temperature rapidly and significantly. The He-3 isotopes are now scattered over a larger volume, and have acquired space to move around and constantly interact with the He-4 atoms. This process effectively uncaps the limitations we priorly believed existed in search of absolute zero - the center of that machine is 2000x colder than Pluto, which was once the "coldest place in existence." Simplified, the heat of mixing effect is not about equalizing the temperature between He-3 and He-4 - it's the opposite. It's about exploiting their differences in heat capacity to render the coldest readings of all time.
Reinterpreting the Rules
We've journeyed into the fascinating world of quantum computing, and explored the fundamentals of the science behind the hype. We've uncovered the profound differences between classical computers and their quantum counterparts, delving into the non-linear realm of quantum mechanics. We've even discovered the surprising need for extreme cold, venturing into the frigid depths of dilution refrigerators. But what does it all mean, actionably? What are the implications of this revolutionary technology for our society, especially in our time?
Comprehensively, quantum computing is not just about building faster computers. It's about fundamentally changing how we solve problems. It's about unlocking a universe of possibilities, from designing life-saving drugs to creating materials with unprecedented properties. It's about confronting problems incapable of being solved through procedural processes. Imagine a world where we can simulate complex molecules spontaneously with pinpoint accuracy, leading to personalized medicines and revolutionary therapies. Imagine a world where we can crack encryption codes that are currently considered unbreakable, revolutionizing cybersecurity and global finance. Imagine a world where we can optimize complex systems, from transportation networks to financial markets, to create a more efficient and sustainable future. The potential is truly boundless. But we're still in the earliest stages of this journey, and as aforementioned, the age of widely-accessible quantum technology is far ahead of us. We face significant challenges, like scaling up quantum computers, developing new algorithms, and overcoming the inherent fragility of qubits. But - with the right investment, innovation, and collaboration, the future of quantum computing is bright as the sun. There are things we could only currently interpret as magic that will be made possible through quantum computing.
Dedication
This piece would not have been possible without the expertise of the brilliant Ciprian Jichici, a leading expert in the field of quantum computing. Thank you so much for all of your time in helping teach me the foundations of quantum technology!
Cobi Tadros is a Business Analyst & Azure Certified Administrator with The Training Boss. Cobi possesses his Masters in Business Administration from the University of Central Florida, and his Bachelors in Music from the New England Conservatory of Music. Cobi is certified on Microsoft Power BI and Microsoft SQL Server, with ongoing training on Python and cloud database tools. Cobi is also a passionate, professionally-trained opera singer, and occasionally engages in musical events with the local Orlando community. His passion for writing and the humanities brings an artistic flair with him to all his work! |
Tags:
- AI (4)
- ASP.NET Core (3)
- Azure (13)
- Conference (3)
- Consulting (2)
- cookies (1)
- CreateStudio (5)
- creative (1)
- CRMs (4)
- Data Analytics (3)
- Databricks (1)
- Event (1)
- Fun (2)
- GenerativeAI (4)
- Github (1)
- Markup (1)
- Microsoft (13)
- Microsoft Fabric (2)
- NextJS (1)
- Proven Alliance (1)
- Python (6)
- Sales (5)
- Sitefinity (13)
- Snowflake (1)
- Social Networking (1)
- SQL (2)
- Teams (1)
- Training (2)
- Word Press (1)
- Znode (1)
Playlist for Sitefinity on YouTube
Playlist for Microsoft Fabric on YouTube
Playlist for AI on YouTube
Copyright © 2025 The Training Boss LLC
Developed with Sitefinity 15.3.8500 on ASP.NET 9